Major research outcome
- Ph.D. Candidate Jaehee Lee and Professor Hansohl Cho, Extreme mechanics of thermoplastic elastomers: Are they rubbers or plastics?
- 관리자 |
- 2023-08-24 17:20:46|
- 97
Polyurethane-urea (PUU) materials are phase-separated copolymers that possess remarkable combinations of mechanical properties of elastomeric and plastomeric materials. The hybrid mechanical features involving both shape recovery and energy dissipation in the PUU materials result from the phase-separating microstructures of hard and soft domains as schematically shown in Figure 1(a). Such hybrid mechanical features in the PUU materials have facilitated their applications in multi-functional soft material architectures ranging from stimuli-responsive polymeric materials to soft robotics and actuation, bio-compatible devices, and impact- or shock-mitigating composites, often subjected to extreme strains and strain rates.
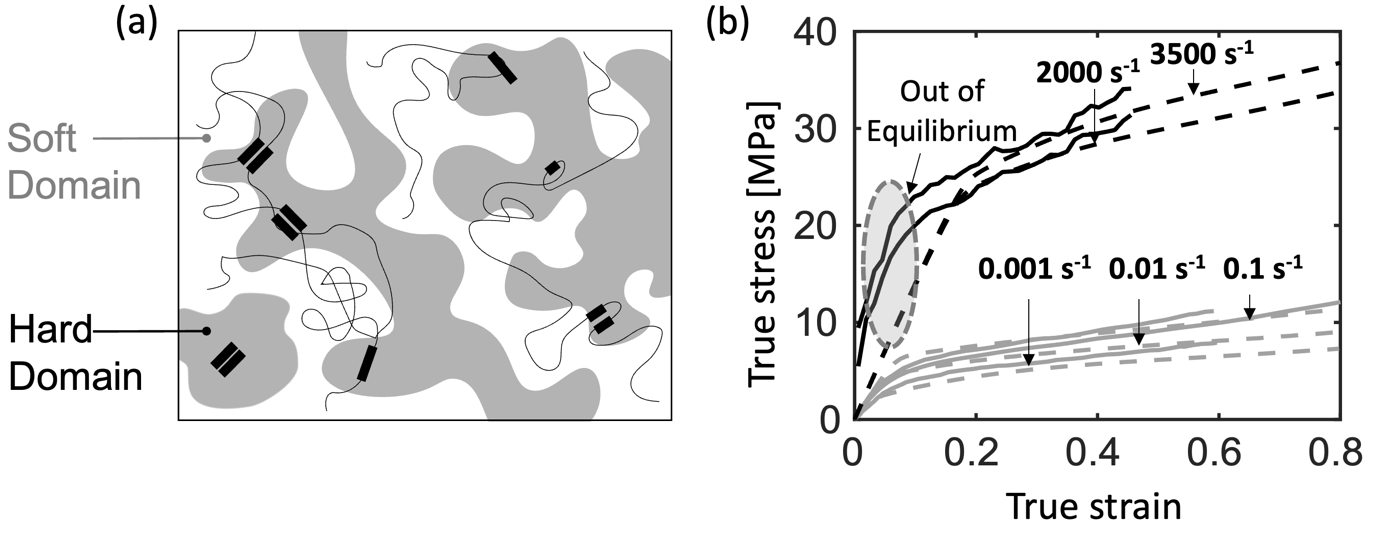
Figure 1: (a) Schematic illustration of the phase-separated microstructure of PUU and (b) stress vs. strain curves at low (0.001, 0.01, and 0.1 s⁻¹) to high strain rates (2000 and 3500 s⁻¹) in compression (solid lines: experiments, dashed lines: model).
In recent work led by Ph.D. candidate Jaehee Lee and Professor Hansohl Cho in the Department of Aerospace Engineering, KAIST, in collaboration with the U.S. Army Research Laboratory (ARL) and Massachusetts Institute of Technology (MIT), a thermodynamically-consistent constitutive theory for the extreme mechanical behavior of the multi-phase polymeric material, PUU, was proposed. The proposed constitutive model comprises multiple micro-rheological mechanisms to account for the individual stress resistances from each of the hard and soft domains that are in “thermoplastic” and “rubbery” states, respectively, at ambient conditions. Numerical simulation results at low (0.001, 0.01, 0.1 s⁻¹) to high strain rates (2000 and 3500 s⁻¹) are displayed along with the experimental data in Figure 1(b). The soft domain provides additional stiffness at high strain rates while the resistance from the soft domain is negligible at low strain rates. Therefore, the mechanical behavior becomes more plastomeric with greater hysteresis and residual strain at high strain rates while it becomes more elastomeric with decreased dissipation and increased shape recovery at low strain rates. The simple yet physically-informed theoretical framework was capable of capturing the hybrid rubbery and thermoplastic features in this important class of soft materials across a wide range of strains and strain rates (or time-scales).
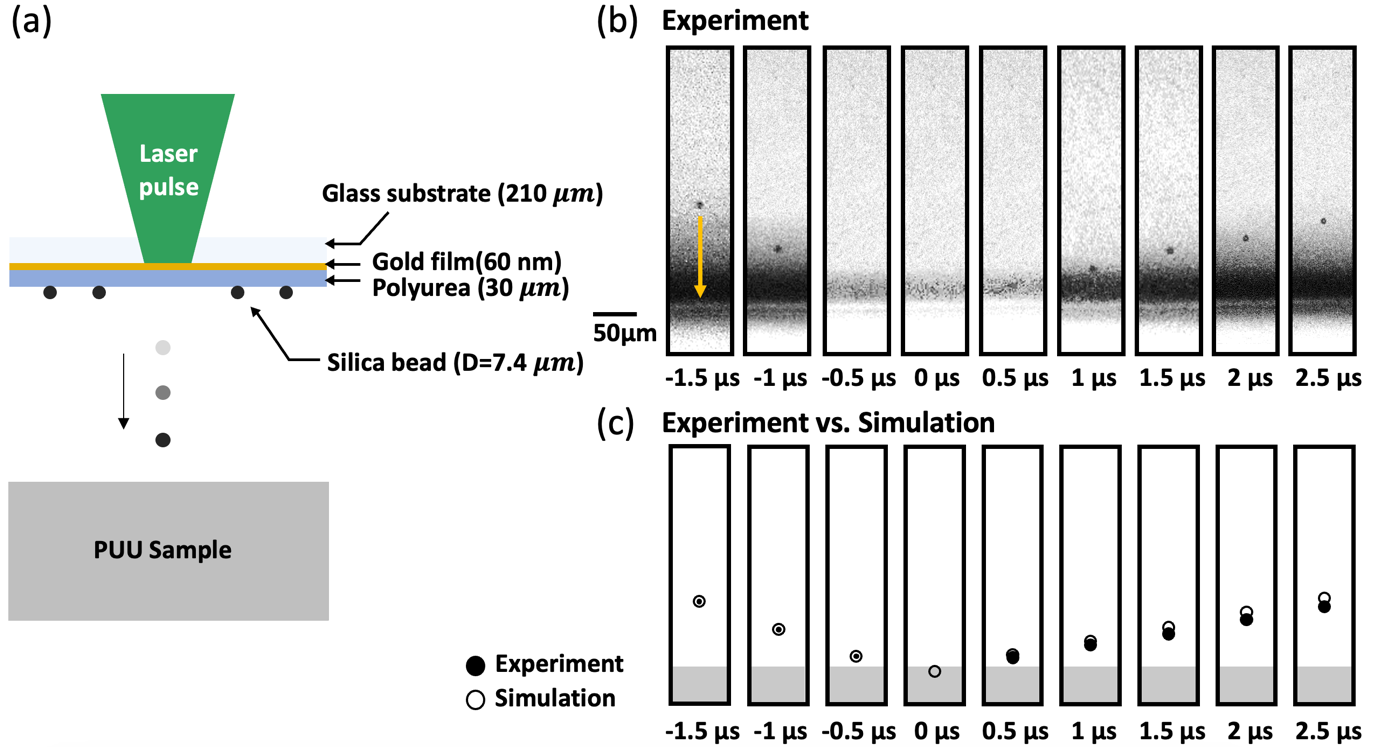
Figure 2: (a) Schematic illustration of the laser-induced particle impact test (LIPIT) and sequential images of the micro-particle trajectories prior to and after impact at 100 m/s in (b) experiment (ARL, MIT) and (c) experiment and numerical simulation (KAIST).
The extreme deformation features were further elucidated by laser-induced micro-particle impact tests (LIPIT) for the PUU materials as illustrated in Figure 2(a), where an ultrafast strain rate (>10⁶ s⁻¹) incurred. Figure 2(b) and (c) show highly asymmetric behavior between the incident and rebound trajectories observed in both experiments and numerical simulations, attributed to significant dissipation processes in the PUU samples under severe impact conditions. The proposed model captured the main features in resilience and dissipation for the strongly inhomogeneous deformation fields at extreme strain rates (>10⁶ s⁻¹) reasonably well.
This work will guide further research for characterizing and harnessing the extreme deformation mechanics of other multi-phase polymeric materials and composites in harsh, multi-physical environments. This work was financially supported by the National Research Foundation of Korea and the U.S. Army Research Office, and recently published in International Journal of Solids and Structures (Vol. 280, 2023; https://doi.org/10.1016/j.ijsolstr.2023.112360).
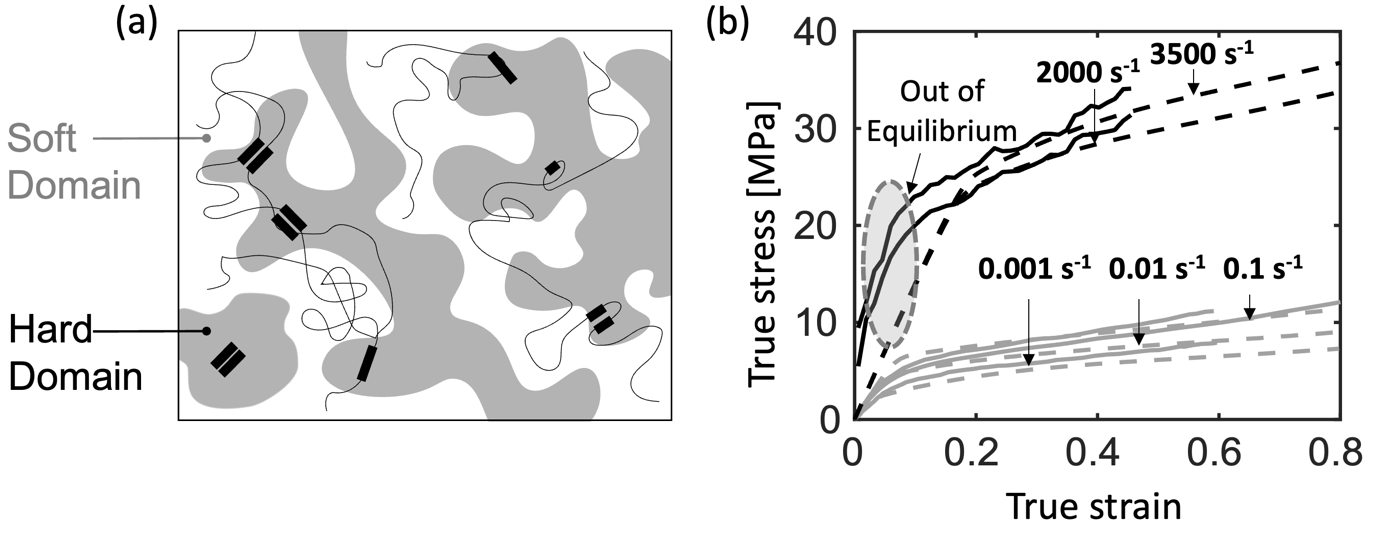
Figure 1: (a) Schematic illustration of the phase-separated microstructure of PUU and (b) stress vs. strain curves at low (0.001, 0.01, and 0.1 s⁻¹) to high strain rates (2000 and 3500 s⁻¹) in compression (solid lines: experiments, dashed lines: model).
In recent work led by Ph.D. candidate Jaehee Lee and Professor Hansohl Cho in the Department of Aerospace Engineering, KAIST, in collaboration with the U.S. Army Research Laboratory (ARL) and Massachusetts Institute of Technology (MIT), a thermodynamically-consistent constitutive theory for the extreme mechanical behavior of the multi-phase polymeric material, PUU, was proposed. The proposed constitutive model comprises multiple micro-rheological mechanisms to account for the individual stress resistances from each of the hard and soft domains that are in “thermoplastic” and “rubbery” states, respectively, at ambient conditions. Numerical simulation results at low (0.001, 0.01, 0.1 s⁻¹) to high strain rates (2000 and 3500 s⁻¹) are displayed along with the experimental data in Figure 1(b). The soft domain provides additional stiffness at high strain rates while the resistance from the soft domain is negligible at low strain rates. Therefore, the mechanical behavior becomes more plastomeric with greater hysteresis and residual strain at high strain rates while it becomes more elastomeric with decreased dissipation and increased shape recovery at low strain rates. The simple yet physically-informed theoretical framework was capable of capturing the hybrid rubbery and thermoplastic features in this important class of soft materials across a wide range of strains and strain rates (or time-scales).
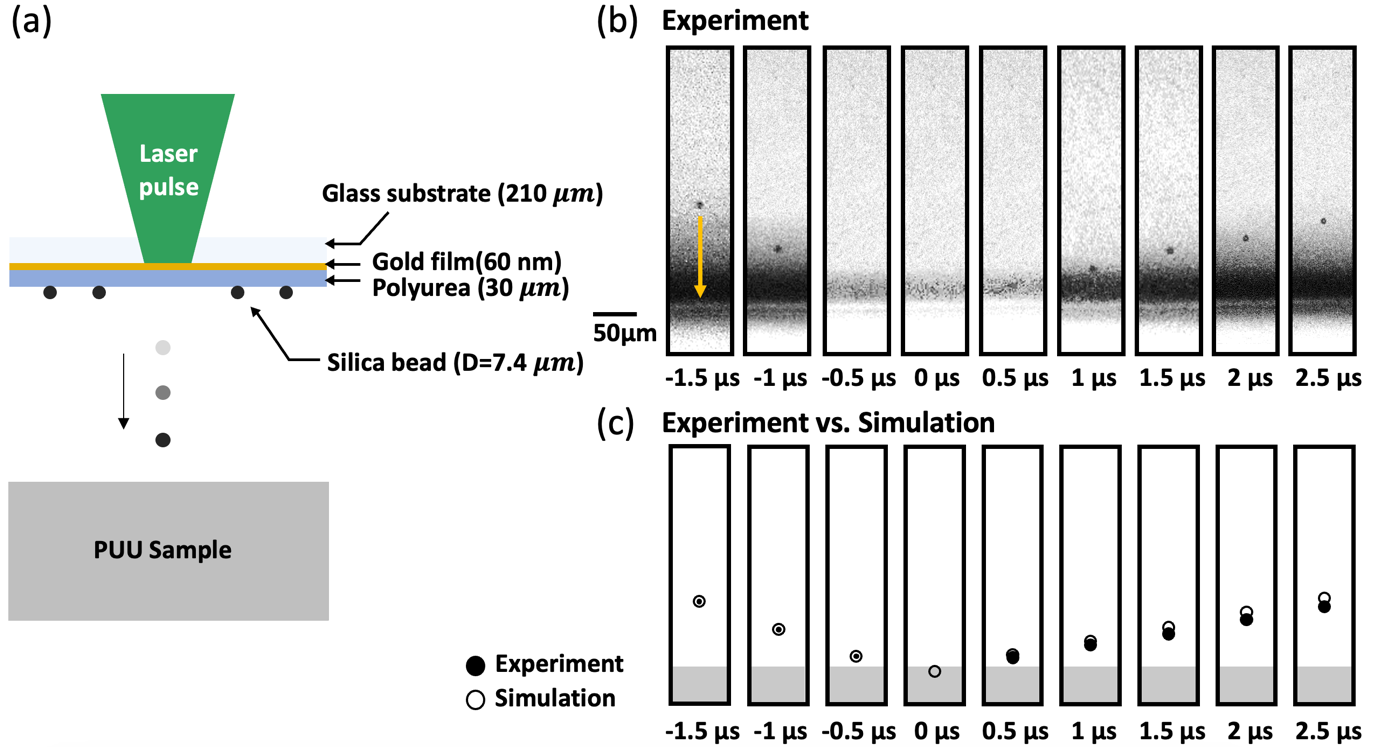
Figure 2: (a) Schematic illustration of the laser-induced particle impact test (LIPIT) and sequential images of the micro-particle trajectories prior to and after impact at 100 m/s in (b) experiment (ARL, MIT) and (c) experiment and numerical simulation (KAIST).
The extreme deformation features were further elucidated by laser-induced micro-particle impact tests (LIPIT) for the PUU materials as illustrated in Figure 2(a), where an ultrafast strain rate (>10⁶ s⁻¹) incurred. Figure 2(b) and (c) show highly asymmetric behavior between the incident and rebound trajectories observed in both experiments and numerical simulations, attributed to significant dissipation processes in the PUU samples under severe impact conditions. The proposed model captured the main features in resilience and dissipation for the strongly inhomogeneous deformation fields at extreme strain rates (>10⁶ s⁻¹) reasonably well.
This work will guide further research for characterizing and harnessing the extreme deformation mechanics of other multi-phase polymeric materials and composites in harsh, multi-physical environments. This work was financially supported by the National Research Foundation of Korea and the U.S. Army Research Office, and recently published in International Journal of Solids and Structures (Vol. 280, 2023; https://doi.org/10.1016/j.ijsolstr.2023.112360).
Attach File |
---|